|
Cycle 2 (2012 Deadline)
Derailing witchweed (Striga) virulence in rice to achieve durable and broad-spectrum resistance PI: Steven Runo (Kenyatta University) U.S. Partner: Michael P. Timko (University of Virginia) Project Dates: August 2013 to January 2016 Evidence to Action Supplement: July 2017- June 2018
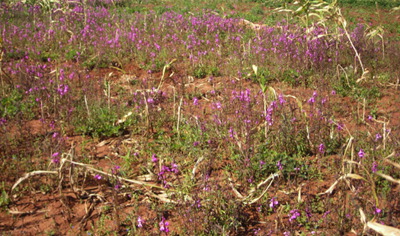 Striga infests two-thirds of arable African land, and constitutes the biggest single biological cause of crop damage in terms of grain yield loss, worth $US 7 billion annually (Photo courtesy Dr. Runo).
| 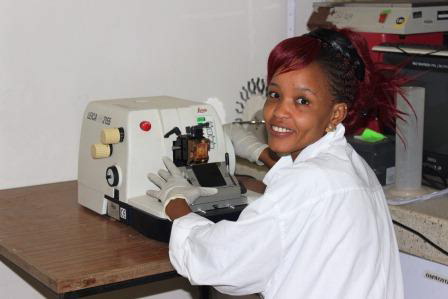 | Ms Dorothy Mbuvi using the microtome in the lab. Photo courtesy Dr. Runo). |
Striga spp. are successful parasitic plants that are notoriously difficult to control mainly because the mechanisms of the biological processes underpinning host-parasite compatibility are poorly understood. Striga affects plant growth very quickly after attaching to the host roots. Within 2-4 days of attachment the crop plants are already visibly stunted. Although the mechanism underlying this early negative effect on crop growth is presently unknown, it has implications for control of the parasite, as control measures need to act before or very shortly after attachment of the parasite to the host. It is now emerging that Striga, like other plant pathogens, produces an array of virulence factors (effectors) that may be allowing the parasite to circumvent and subdue the host defense. The long-term goal of the researchers conducting this project is to identify mechanisms controlling release of these virulence factors as a first step toward developing breeding strategies that can be used to build durable resistance to Striga hosts. The specific aims of the current project are designed to provide a comprehensive assessment of the mutations or polymorphisms in Striga effectors as well as their effect in plant cells. The project should result in the identification of various races of Striga for effector genes and their role in virulence, which is of fundamental importance to understanding the molecular nature of the plant-plant resistance interactions. An output of this project will be identification of multiple factors (effectors) that help Striga evade resistance by its host. An additional output will be quantification of how these factors are able to change with time and acquire ability to invade new hosts. It is hoped that the specificity of different Striga virulence races (ecotypes) to different host cultivars will be identified. This knowledge can then be directly applied to breeding of new cultivars resistant to Striga, through gene pyramiding. Because yields of some of the most important crops in Africa, including rice, corn, millet, and sorghum, are being reduced due to the impact of Striga, the results of this project could have a significant impact on agricultural productivity in this region and others where the parasitic plant is a problem. Final Summary of Project Activities This project ended in September 2016 before receiving a supplement in 2017 to disseminate the results further. Below are the main highlights of the research.
Novel Sources of Striga Resistance from Wild Sorghum Accessions Identified: The team sought to determine to what extent wild sorghum are resistant to Striga. The screening strategy involved controlled laboratory assays on rhizotrons – where they artificially infected sorghum with Striga – as well as field experiments in three sites where they grew sorghum under natural Striga infestation. They tested the resistance response of 7 accessions of wild sorghum of Aethiopicum, Drummondii and Arundinaceum races against N13, a cultivated Striga resistant landrace. From the laboratory experiments, they found three wild sorghum accessions (WSA-1, WSE-1, and WSA-2) that had significantly higher resistance than N13. These accessions had the lowest Striga biomass, fewest and smallest Striga attached to them. Further microscopic and histological analysis of attached Striga haustorium showed that wild sorghum accessions either hindered ingression of Striga haustorium into the host cortex or induced intense hypersensitive reaction (HR) at the infection site, a hallmark of systemic acquired resistance (SAR). Field experiments affirmed the laboratory screening experiments where these same accessions were found to have resistance against Striga. In the field, the wild sorghum had low Area under Striga Number Progressive curve (AUSNPC) – which measures emergence of Striga from a host over time. They concluded that wild sorghum accessions are an important reservoir for Striga resistance that could be used to expand the genetic basis of cultivated genotypes for resistance to the parasite.
Strigolacton Profile of Wile and Cultivated Sorghum Determined: There is yet another important aspect of Striga resistance, germination stimulant (strigolactone) production, which provides a level of resistance on host crops due to decreased strigolactone (signaling molecules produced by plants) production results in lower frequency of Striga germination. The study sought to determine SL production in wild sorghum accessions as these have been found to be tolerant to Striga infestation. The study further determined the relationship between SL production and tillering in wild sorghum accessions. Experiments involved collection of root exudates from sorghum and using them to induce Striga germination. Liquid chromatography-tandem mass spectrometry was used to determine the type and amount of SL in wild sorghum root exudates. Results showed that: i) the most abundant SL in wild sorghum was 5-deoxystrigol, ii) wild sorghum contained low levels of SL compared to their cultivated counterparts, 5-deoxystrigol has been reported earlier as stable strigolactone molecule making plants producing it more susceptible to Striga and this could explain why the wild cultivars though producing very low amounts of 5-deoxytrigol were able to induce germination iii) there is an inverse relationship between strigolactone concentration and tillering and a positive relationship between the Strigolactone concentration and Striga germination stimulation. All sorghum genotypes were able to induce Striga germination even in genotypes that produced least amounts of 5-deoxtrigol. These genotypes could provide more insights in developing sorghum lines that are resistant to Striga weed.
Striga Sorghum Arms Race as Revealed by Dual RNA Sequencing: The team then carried out dual RNA sequencing (both host and parasite tissue) of wild and cultivated sorghum and their parasites, using Illumina Hiseq. RNA sequencing data was used to determine genes in wild sorghum that are differentially expressed at early and late S. hermonthica infection and compared their profile to those of cultivated sorghum. In addition, they compared the profile of differentially expressed genes between S. hermonthica infecting wild and cultivated sorghum. Finally, the team also compared the profile of differentially expressed genes between S hermonthica and S. asiatica in cultivated sorghum. They found that more genes were differentially expressed in wild sorghum upon Striga infection and that more Striga genes were differentially expressed when the infection was on wild sorghum. These findings suggest that wild sorghum has more genetic diversity to cope with Striga and that more genes in Striga are required to overcome resistance from the host. RNA sequencing data further led them to identify a set of candidate Striga resistance genes from wild sorghum as well as a rapporteur of molecules (effectors) that may be aiding Striga to overcome the innate immunity of its hosts Lastly, unlike many plant-pathogen interactions, little is known about the factors that control the association of parasitic plants with their host. Using RNA sequencing, the team profiled differentially expressed genes in sorghum and in Striga. They then developed a model that can predict protein-protein interactions (PPI) between Striga and sorghum, and developed a platform for Striga genetic transformation in order to validate the interactions. For identification of interacting proteins, a database for parasite-host protein-protein interaction was created from existing literature. Predictions of the interactions were done based on protein domain data, homology, and gene ontology terms, in addition to the interaction databases. To identify interacting Striga and sorghum proteins a machine learning approach, Support Vector Machine (SVM) classifier, was applied. The learning algorithm was trained to predict protein-protein binding sites from inter-domain interactions while still distinguishing interacting and non-interacting proteins. The project team has identified sorghum lines that have resistance to Striga. They will develop this further to lines that farmers can grow in the short term. They have also identified potential effectors 'what Striga uses to overcome host resistance'. These will be further characterized and used to 'derail Striga' in its virulence. Through this PEER project, three students were trained at Master of Science level. Two of these have submitted their thesis for examination. Also, due to the role of women in agricultural value chains and well documented evidence of technology uptake when women are involved, the project specifically trained two women PublicationsRuno S, Kuria, E. Habits of a Highly Successful Cereal Killer, Striga. PLoS Pathogens, 2018. 14(1): e1006731. DOI: https://doi.org/10.1371/journal. ppat.1006731. Mbuvi, D. A., Masiga, C. W., Kuria, E., Masanga, J., Wamalwa, M., Mohamed, A., Timko, MP, Runo, S. Novel Sources of Witchweed (Striga) Resistance from Wild Sorghum Accessions. Frontiers in Plant Science, 2017 8, 271. DOI: https://doi.org/10.3389/fpls.2017.00116. Back to PEER Cycle 2 Grant Recipients
|
|
|
|